Transcription in eukaryotes is more complex than transcription in prokaryotes. Following are the reasons for its complexity:
Genome size: Eukaryotic genomes have many genes for recognition and transcription as compared to prokaryotes.
Presence of introns: Eukaryotes possess introns (non-coding DNA sequence) and exons (coding DNA sequence). Due to the presence of introns, the distance between two genes becomes larger and that creates complexity. For instance, in E. coli (prokaryotes), the number of genes per million base pairs is 900 whereas in drosophila (eukaryotes), it is just 110 genes.
And in case of humans, it is only 9 genes per million base pairs. This density makes transcription initiation a complicated process. Besides, in the genomes of multicellular organisms, finding the start of a gene is like finding a needle in a haystack.
Types of RNA polymerases in Eukaryotes
Due to the presence of several complexities in eukaryotic genome – they have divided the job of transcription in eukaryotes among three different RNA polymerases:
- RNA polymerase I – transcribes rRNA (except 5s rRNA)
- RNA polymerase II – transcribes all protein-coding genes for which the ultimate transcript is mRNA (i.e., heterogenous nuclear RNA — hnRNA)
- RNA polymerase III – transcribes small functional RNA (SnRNA) + tRNA + 5s rRNA
Note: In eukaryotic transcription, we’ll focus on RNA polymerase II only.
We have already discussed transcription in prokaryotes, where sigma factor is required for the recognition of the consensus sequence on the DNA coding strand; and once transcription factor begins, the sigma factor dissociates from the RNA polymerase and the core polymerase enzyme continues to synthesize RNA within a transcription bubble that moves along the DNA.
It is important to mention that in bacteria – RNA polymerase holoenzyme is equal to core enzyme plus sigma factor. Once the sigma factor is removed from the holoenzyme, the remaining part is called the RNA polymerase core enzyme.
Similarly, in eukaryotes, the core of the RNA polymerase II cannot recognize promoter sequence on its own. Though in bacteria, sigma factor is an integral part of the holoenzyme – GTF’s (General Transcription Factor) in eukaryotes is not an integral part of the RNA pol II rather it is independent of the enzyme, which is required to bind to regions on the promoter before the binding of the core enzyme. GTFs are proteins that do not take part in transcription, but binds to the sequence of the promoter to attach RNA polymerase II at the correct site to start the transcription process.
GTFs are of the following
types:
-
- TFII D
-
- TBP
-
- TFIIB
-
- TFIIF
-
- TFIIE
-
- TFIIH
MNEMONICS TO REMEMBER
TRANSCRIPTION FACTOR IN SEQUENCE
Dogs Bark
and Bite People Frequently Every Halloween
Dogs = TFIID; Bark = TBP; Bite = TFIIB; People = RNA P; Frequently = TFIIF; Every = TFIIE; Halloween= TFIIH
Initiation Process of Transcription in Eukaryotes
STEPS:
- GTF’s (6 factors) which do not take part in RNA synthesis, recognize and bind to sequences in the promoter or to one another to attract the RNA polymerase II core and position it at the correct site to start transcription.
- First the TFIID bind to the TATA box (HOGNESS BOX) which is at -30bp upstream. The TFIID has a binding site TBP (TATA Binding Protein) that attaches to the TATA box. This attracts other factors and enzymes to bind to the DNA.
- After that TFIIB binds to form a TFIIB-TBP complex
- After that TFIIF and RNA polymerase bind to the promoter
- Finally, TFIIE and TFIIH bind to the site (TFIIH has DNA helicase activity that promotes the unwinding of DNA near the RNA start site – a process requiring the hydrolysis of ATP), thereby creating an open complex. TFIIH has an additional function during the initiation phase.
- A kinase activity in one of the subunits phosphorylates pol II at many places in the CTD (it is a protein tail of beta subunit of RNA polymerase II, called the Carboxyl Tail Domain). This causes a conformational change in the overall complex, initiating transcription. Phosphorylation of the CTD is also important during the subsequent elongation phase, and it affects the interactions between the transcription complex and other enzymes involved in processing the transcript.
- When all GTF’s and RNA polymerase bind to the DNA – they form a PREINITIATION COMPLEX.
- Once transcription initiates – RNA Polymerase II dissociates from most of the GTFs to elongate the primary RNA transcript. However, some factors remain attached to the enzyme to attract the next RNA polymerase core. In this way, multiple RNA polymerase II enzymes can be synthesizing transcripts from a single gene at one time.
- During synthesis of the initial 60-70 nucleotides of RNA, first TFIIE and then TFIIH is released and pol II enters the elongation phase of transcription.
ELONGATION of Transcription in Eukaryotes:
- Elongation takes place inside the transcription bubble essentially the same way as for the synthesis of prokaryotic DNA. However, nascent RNA has very different fates in prokaryotes and eukaryotes. In prokaryotes, translation begins at the 5’-end of the nascent RNA while the 3’-half is still being synthesized.
- TFIIF remains associated with pol II throughout elongation. Once RNA transcript is completed, transcription is terminated. RNA Polymerase II is dephosphorylated and recycled, ready to initiate another transcript. The elongation of RNA by RNA polymerase in both bacteria and eukaryotes is inhibited by the antibiotic ACTINOMYCIN D, RIFAMPICIN, etc.
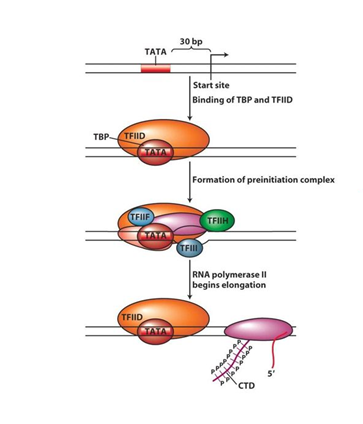
In the past, it was thought that most of the processing of pre-mRNA takes place after RNA synthesis is completed. Processing after RNA synthesis is called Post-transcriptional processing. Recent studies, however, showed that processing actually takes place during RNA synthesis. This is called Co-transcriptional processing.
The CTD of RNA polymerase II plays a significant role in coordinating processing events. The CTD is composed of many repeats of a sequence of seven amino acids. These repeats serve as binding sites for some of the enzymes that are required for RNA capping, splicing, and cleavage followed by polyadenylation.
The CTD is located near the site where nascent RNA emerges from the polymerase so that it is an ideal place to orchestrate the binding and release of proteins needed t process the nascent RNA transcript while RNA synthesis continues. Phosphorylation and dephosphorylation of CTD determines which processing proteins can bind.
RNA of eukaryotes undergoes further
processing before it is translated. RNA processing includes:
- Addition of 5’cap and 3’ tail to the nascent RNA.
- The second processing involves RNA splicing i.e., the removal of introns. The removal of introns and the joining of exons is called SPLICING.
POST TRANSCRIPTIONAL MODIFICATION
- 1. Capping: at the 5’-end, there is addition of 7-methyl guanosine triphosphate. However, in the beginning, only GTP is added and later on, methyl group is added. This reaction is catalyzed by the enzyme guanyl transferase. And for the transfer of methyl group – methyl transferase enzyme is utilized. Till the time this capping is being done, the RNA is released from the RNA polymerase.
- 2. Tailing: this is done by adding 200-300 adenylate residue; and for this addition ATP is used as a substrate in the presence of the enzyme adenylate polymerase. It is important to note that the tailing is nothing but the formation of additional RNA as nucleotides are being added to the RNA. However, this RNA formation is not dependent on DNA and thus called as Template Independent RNA synthesis.
- 3. Splicing: for this process, a protein complex is required, called SPLICEOSOME / SNURPS / SnRNP (small nuclear ribonucleo protein). The SNURPS are responsible for the formation of intonic loop or lariat. Finally, the introns are removed and all exons are joined together by RNA ligase. This leads to the formation of messenger RNA (mRNA).

The conserved nucleotides in the transcript on the intron are recognized by small nuclear ribonuceloprotein particles (SnRNPs), which are complexes of protein and small nuclear RNA. In other words, proteins and SnRNA combined to form SnRNP. A functional splicing unit is composed of a team of SnRNPs called SPLICEOSOME.
Self splicing and RNA world
Though splicing is done by SPLICEOSOMES in 1981, Tom Cech and coworkers reported that in a test tube, the primary transcript of an rRNA from the ciliate protozoan Tetrahynema can excise a 413 nucleotide intron from itself without the addition of any protein. Subsequently, other introns have been shown to have this property and they have come to be known as SELF-SPLICING INTRONS.
This finding is considered a landmark discovery in biology because it marked the first time that a biological molecule other than protein was shown to catalyze a reaction. This discovery and similar other ones have provided solid evidence for a theory called the RNA WORLD, which holds that RNA must have been the genetic material in the first cell because only RNA is known to both encode genetic information and catalyze biological reactions.